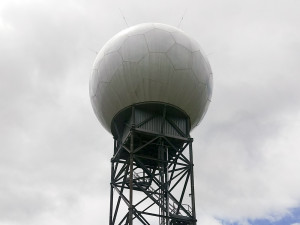
You probably take radar for granted. It’s just one of those commonplace, everyday technologies that you interact with—one way or another—all the time. When there is severe weather in your area, the television weathermen and online sources all put familiar Doppler radar images front and center. We know when and where it will be raining or snowing, can estimate how much precipitation we’ve received, and can even track wind patterns, determine if a tornado is forming, and issue critical warnings before they strike.
Weather radar is the kind that we ‘regular folks’ interact with all of the time, but it’s not the only kind. Police departments use car-based and handheld radar systems to find and catch speeders, which may or may not be a good use of their time (and our money). Scientists can use ground-penetrating radar systems to study the Earth’s crust. Boat- and ship-based marine radar systems are an essential part of navigation and collision avoidance, especially in poor weather, and in many harbors the ‘vessel traffic services’ use radar (and other) systems to manage traffic and improve safety.
Radar systems, small and large, also form an integral part of our aviation network. The air traffic control system relies on radar (combined with other technologies) to keep track of the aircraft overhead and help ensure that they reach their destinations safely. Controllers can guide aircraft away from ground obstacles and each other, and give instructions that lead pilots to their intended destinations even in the most adverse of conditions.
In addition to these ground-based systems, commercial aircraft have multiple radar systems operating on-board the aircraft itself. Since 1965, commercial aircraft operating in the United States have been required to have on-board weather radar systems (14 CFR 121.357). And in the aftermath of the 1974 crash of TWA Flight 514 (see a previous article in this series), they were also required to have a ground proximity warning system (39 FR 44439). These proximity warning systems have been implemented in the form of a radar altimeter . . . a device that, using radar, monitors and reports to the pilots the actual distance between the aircraft and the ground, and sounds warnings when the plane is too low.
There are two important radar sites located in Loudoun County, Virginia, and one more located about seven miles beyond our borders in Fauquier County, Virginia.
The History of Radar

Radar is a technology that can determine the distance, angle, or velocity of objects through the use of radio waves, and so its development closely parallels our expanding knowledge of radio.
Heinrich Hertz, a German physicist, proved the existence of electromagnetic waves in a series of experiments in the 1880s. During these experiments, Hertz transmitted and received radio pulses . . . and also discovered that radio waves would reflect off surfaces, especially metallic surfaces, much like light can be reflected and modified when it comes in contact with objects. In honor of Hertz’s work, the most commonly used unit of frequency is named in his honor; a Hertz is equal to one cycle per second.
Another nineteenth century pioneer, Guglielmo Marconi of Italy, took an interest in electricity and radio waves. When Hertz died in 1894, the renewed public attention to his discoveries prompted Marconi to begin developing a ‘wireless telegraph’ system. Similar systems had been developed by others, but none were successful, and none were able to transmit further than about a half kilometer. In less than a year, Marconi had developed both a lightning detection system and a short-range wireless telegraph similar to those developed by others. By the summer of 1895, he had improved his system so that it could transmit more than two kilometers—likely a record at the time—and had come to believe that, given sufficient funding, he could develop and commercialize a system that could transmit signals over great distances.
Failing to develop interest (or funding) in Italy, Marconi—then only twenty-one years old—moved to England in 1896 and quickly made contacts in the Admiralty and the British Post Office. He soon delivered a series of public lectures and demonstrations of his inventions. Marconi’s efforts began to receive international attention, and he even traveled to the United States to demonstrate radio transmission. In 1902—only eight years after he began to research radio in earnest—Marconi sent the world’s first successful radio message across the Atlantic Ocean. By 1904 a commercial Marconi service was transmitting nightly news summaries to ocean liners in the Atlantic, and by 1907 a service was transmitting radio-telegraph messages across the Atlantic.
One of Marconi’s discoveries would later become the fundamental basis of radar. In 1899, while testing a radio beacon system on the Salisbury Plain in England, he discovered that radio waves were being reflected back to the transmitter. Marconi noted that this could be useful if applied at lighthouses and lightships, and could enable ships to locate (and avoid) dangerous areas, but he was focused on improving communication radio systems and did not further develop the idea.
In 1904, a German inventor named Christian Hülsmeyer gave public demonstrations of a ship-based precursor to modern radar. His system would use a directional antenna to send out a radio signal, and a receiver to detect that signal being reflected back. At a range of about three kilometers, this system could effectively detect the presence, and direction toward, other nearby ships. Hülsmeyer proposed mounting the system, which he called the ‘telemobiloscope,’ on a high mast and spinning it around to paint a sort of picture of what surrounded the ship. He planned to expand its range to ten kilometers, and had also developed a triangulation system by which an operator could [manually] estimate the distance to a return . . . but officials in Germany were uninterested in the system, and it never went into commercial production.
Many systems like Hülsmeyer’s were developed in the decades following, but none gained widespread use or acceptance. Although they were reasonably capable of determining an object’s general direction, they were unable to provide reliable distance information except through painstaking, manual calculations.
It wasn’t until the 1930s, with the development of pulsed radio signals and reliable oscilloscopes, that it became possible to reliably measure the distance of an object. By this time, the military applications of the technology were clear, and many countries had active, secret programs working to develop it. Between 1934 and 1939, eight nations—France, Germany, Italy, Japan, the Netherlands, the Soviet Union, the United Kingdom, and the United States—had all independently developed systems that would today be considered true [though rudimentary] radar systems. By the end of the Second World War in 1945, Australia, Canada, Hungary, New Zealand, and South Africa had all created systems as well.
The term ‘radar’ was coined by the United States Navy around 1940. It was originally an acronym that meant RAdio Detection And Ranging, but has since become a word in its own right. The Navy’s development program had entered sea trials in 1939 with a system designed to detect ships and aircraft. Deliveries of a full production version, the CXAM system (built by RCA), began in 1940 and were rapidly installed on Naval vessels. The United States Army was developing their own system simultaneously, and the first prototype of their SCR-268 system was tested at Fort Monroe, Virginia, in 1938. Westinghouse began delivering improved production versions—the SCR-270 and SCR-271—to the Army in 1940.
Five of these early SCR-270 radar systems were deployed by the Army on the island of Oahu, Hawaii. On the morning of December 7, 1941, the operators of the radar located at Opana Point on the northern tip of Oahu detected a large formation of aircraft and radioed it in to the intercept center, where it was dismissed as a formation of six U.S. B-17’s returning from the mainland. They had in fact detected the massive formation of Japanese aircraft approaching Oahu, and their attack on the Navy base at Pearl Harbor began about an hour later . . . demonstrating that radar, if used correctly, could be used to detect attacks before they occur.
In 1842, nearly a century earlier, Austrian physicist Christian Doppler postulated the phenomenon now known as the Doppler effect. He had developed the theory as a way to explain the colored light (electromagnetic waves) observed in binary star systems, but it also explained the behavior of other kinds of waves. Due to the Doppler effect, if something is moving toward you, its wave emissions will be received at a higher frequency. If it is moving away from you, its wave emissions will be received at a lower frequency. This explains why the sound of a passing car changes from a higher pitch as it approaches to a lower pitch as it passes by and departs.
Soon after radar technology entered use, scientists and researchers were able to use the Doppler effect to filter out stationary targets, which would reflect at roughly the same frequency that was broadcast. Moving objects would increase or decrease the reflected frequency. This allowed them to get clearer signals over a greater range, all while using less power. The early filtering technology was bulky and unreliable, and it required vibration damping, so it was generally only effective in a fixed location. Doppler-filtered radar would not be practical for use on aircraft and other moving vehicles until decades later.
The Doppler effect also made it possible—after better filters and analysis tools were developed—to determine the speed of a target relative to the radar site, in addition to its direction and distance. This technology eventually developed into modern pulse-Doppler systems, which send microsecond-long pulses of radio signals out, and then precisely measure reflections back for about a millisecond after, and then repeat the process. Using these precision measurements, the size, position, and speed of targets [relative to the radar station] can be measured and displayed to radar operators. And by monitoring the object over multiple pulse cycles, the direction of travel and actual speed [relative to the Earth] can also be calculated and displayed.
NEXRAD and TDWR Weather Radar Systems
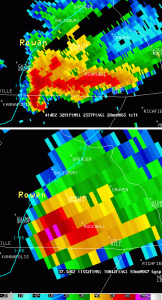
Early radars were very effective at tracking ships and aircraft, but suffered from weather-related interference. Rain and snow returns could degrade—or mask entirely—returns from aircraft. This became a ‘useful bug,’ however, and led to the development of early weather radar systems. In the United States, the first weather radar network was designed in 1957 (the WSR-57 network) and began being deployed by the National Weather Service (NWS) in 1959.
Doppler technology was still largely classified, and so our first civilian weather radar network relied on the early pre-Doppler technology that could provide no information about velocity. The system was difficult to operate even in ideal conditions, and because it was incapable of measuring speed it was essentially useless for tornado prediction. It was only capable of showing precipitation intensity, which could provide valuable hints about where severe weather may occur, but not with the kind of certainty that we have today.
The U.S. National Severe Storms Laboratory (NSSL), established in 1964, experimented with different weather radar systems. They obtained a surplus U.S. Air Force ten centimeter pulse-Doppler radar in 1969, and in 1973 they used it to to clearly document the entire life-cycle of a tornado for the first time. They continued to experiment with Doppler technology, but actual adoption for general forecasting was very slow.
The NWS did begin to deploy an improved weather radar system, the WSR-74 network, in 1973 . . . but this network was also based on pre-Doppler technology, and it coexisted with (instead of replacing) the existing WSR-57 network. Some WSR-57 radars were replaced with WSR-74 radars when they failed, while other WSR-74 systems were placed to fill gaps in the existing network. Although the WSR-74 systems were easier to operate and more reliable, they suffered from the same key shortcoming that the WSR-57 system had: an inability to measure velocity. Incredibly, parts of the combined WSR-57 and WSR-74 network remained in operation until the last site was finally decommissioned in 1999.
In 1976, only a few short years after the WSR-74 deployment had begun, the NSSL established a project to formally study Doppler radar systems as the basis of an improved severe weather detection system . . . but progress still remained absurdly slow. In 1979, a program office was established to move forward designing and deploying a Doppler weather radar system to be called ‘NEXRAD’ (NEXt-Generation RADar), which would be a joint effort of NWS, the Air Force Weather Agency, and the Federal Aviation Administration (FAA). Systems were tested throughout the 1980s, but the final prototype of the new radar—the WSR-88D—did not enter test operation until 1990. The first WSR-88D radar to be put in forecast operation was the one installed at the NWS office in Sterling, Virginia—right here in Loudoun County—in 1992.
The WSR-88D radars that make up the NEXRAD network have a ten centimeter wavelength, a beam width of 1.25 degrees, can measure velocity accurately up to sixty-two knots, and have a range of about 230 kilometers. There are 160 NEXRAD sites in operation across the United States, which cover most of the continental United States and Hawaii, and the most populated areas of Alaska. They are now one of the most useful forecast tools used by NWS for detecting—and warning about—severe weather. Being Doppler-based, they can detect the wind rotation that indicates the possible development of a tornado, and countless lives have likely been saved by the early warning capabilities of the WSR-88D radars.
Although the FAA is one of the partner agencies in the development and deployment of the WSR-88D, the NEXRAD network was not able to meet all of their needs. The range and accuracy of the WSR-88D is excellent, but it falls short when it comes to measuring near-ground wind-shear and down-bursts, and its relatively low resolution doesn’t provide the level of detail that is sometimes needed around airports. So, as the NEXRAD network began to be deployed, the FAA was also working with the Lincoln Laboratory at the Massachusetts Institute of Technology to develop a supplemental radar system that could be deployed near major airports.
This effort resulted in the Terminal Doppler Weather Radar (TDWR) system, which has since been deployed at sixty-four sites across the United States. One is located in Leesburg, Virginia—also right here in Loudoun County—to serve Washington Dulles International Airport. TDWR radars have a five centimeter wavelength (half that of the WSR-88D) and a beam width of only 0.5 degrees (sixty percent narrower than WSR-88D), which results in much more detailed resolution (see comparison image above). However, it can only measure velocity accurately up to about twenty-five knots, and has a very limited range—only ninety kilometers, or sixty percent less than the WSR-88D.
Although the TDWR network is an amazing safety tool and is very valuable to air traffic controllers, it is much less useful than the WSR-88D as a general-purpose weather radar, particularly due to its limited range and the lesser number of sites. However, the NWS does have access to TDWR data, and can use them as an additional forecast tool when necessary.
FAA and USAF Joint Surveillance System
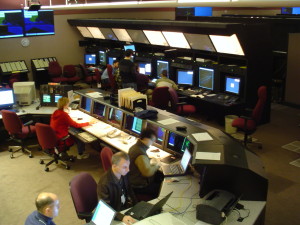
During the Second World War, the U.S. Army established a network of radar stations in the United States to defend against enemy attacks. After the war ended, that emergency system had dwindled until only five of these radar defense stations were still operating. As the realities of the Cold War began to set in, military planners realized that it would be necessary to establish a much more expansive radar defense system to defend against the threat of the Soviet Union and its allies. Several plans were developed, but then abandoned before implementation due to cost or other concerns.
Finally, in 1947, the U.S. Air Force’s Air Defense Command (ADC) went ahead with a stop-gap radar defense network called ‘Lashup’ that expanded the five-radar network already in place. The Lashup net was completed in 1950, and consisted of forty-four radar sites that would constantly monitor U.S. airspace for enemy intrusion. As the temporary Lashup network was completed, the U.S. Air Force finally prioritized and moved forward with a permanent system—which they creatively named the Priority Permanent System. The Permanent system consisted of seventy-five radar sites, and was completely operational by mid-1952. Another 107 ‘gap-filler,’ semi-mobile, and Canadian expansion sites were added before the system was (again) replaced in 1957.
Beginning in 1957, the ADC began implementing a cutting-edge radar and threat management system called the Semi-Automated Ground Environment (SAGE) which would soon replace the [obviously not-very-permanent] Permanent system. By 1963, ADC was managing a complex network of 142 primary and 96 ‘gap-filler’ annex radar sites all across the United States, Canada, Greenland, and Iceland. This system remained in-place until 1980, and was famously controlled by two of the largest computers ever built (which were the most powerful in the world when built, but badly obsolete in 1980).
Meanwhile, the Federal Aviation Administration (FAA) was building their own radar network for managing U.S. airspace. Many airports had radar systems on-site for monitoring the surrounding airspace, but there was no national network for monitoring long-haul flights and it would not be uncommon for flights to be entirely un-monitored while en-route. A new network of Air Route Surveillance Radars, starting with the Raytheon-built ARSR-1, began entering operation in 1958. By 1963 most of the U.S.’s airspace was being actively monitored by the FAA. Later in the 1960s, an improved ARSR-2 entered service. Until 1980, these 1960s-era radar systems remained the backbone of the air traffic system. Some ARSR-1 and ARSR-2 radars are still operational today under the replacement JSS radar network (see below), but they are scheduled for replacement in the next year or two.
At some point in the 1970s, it became evident that the Air Force and FAA were duplicating their efforts. For example, until 1980, the Washington, DC, metropolitan area was monitored by the Aerospace Defense Command (the renamed Air Defense Command) via a SAGE radar site at Fort Meade, Maryland, and by the FAA via an ARSR site in Suitland, Maryland. Although the two radars had different designs, entered service at different times, and were staffed by officials from different agencies, they actually served the same purpose: monitoring the airspace in and around Washington, DC. They had similar ranges and similar capabilities.
This realization led to the development of the Joint Surveillance System (JSS), a unified network of radar sites that would serve the needs of both the FAA and the ADC. The system began operation in 1980, quickly supplanting both the SAGE and the ARSR systems. In areas that were covered by only one radar or where the FAA and ADC radars were already co-located, those single sites became part of the JSS system. Areas that were covered by completely separate SAGE and ARSR radars had their sites combined at one of the two locations, or moved to a new unified location.
Still today, some of the radar sites are running equipment from the earlier SAGE and ARSR systems, although many were upgraded in the 1980s to improved Westinghouse-built ARSR-3 systems, or in the 1990s to Northrup-Grumman-built ARSR-4’s. There are about 106 operational JSS sites today, which are unmanned sites maintained and operated by the FAA. JSS radar data is fed directly to both the FAA and the ADC. Here in the Washington, DC, metropolitan area, we are now served by a single radar site located in The Plains, Virginia (Fauquier County, about seven miles south of the Loudoun border). This site replaced both the Fort Meade and Suitland, Maryland, sites.
LWX Weather Radar (NEXRAD) in Sterling, Virginia
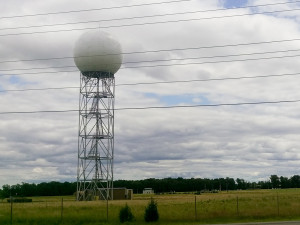
The LWX weather radar became operational in 1992, and was the first WSR-88D site to be placed in forecast operation. It is located in eastern Loudoun County at the Sterling office of the National Weather Service (NWS), adjacent to the northern edge of Washington Dulles International Airport.
The radar dome is clearly visible from nearby Old Ox Road (route 606).
Coming from route 28, proceed west on Old Ox Road (route 606) for about 4.5 miles. The NWS facility, and the LWX radar dome, will be on your left.
Coming from U.S. 50, proceed north on Loudoun County Parkway (route 606), which becomes Old Ox Road, for about four miles. The NWS facility, and the LWX radar dome, will be on your right.
The dome itself is on secured government property and cannot be accessed by the public. There is a small pull-off (the future location of a road) on the north side of route 606 almost immediately across from the radar, which is the best location to stop for a look. Do not attempt to enter the NWS property.
IAD Weather Radar (TDWR) in Leesburg, Virginia
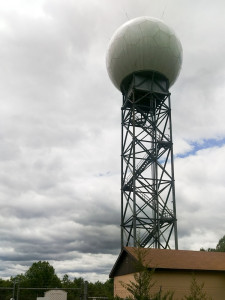
The IAD weather radar is the Federal Aviation Administration’s (FAA) TDWR site serving Washington Dulles International Airport. It is located atop a hill to the southeast of Leesburg, Virginia, in central Loudoun County.
The radar dome is clearly visible at some distance from Harry Byrd Highway (route 7). It can also be visited close-up.
Coming from route 7, exit south on Crosstrail Boulevard. Turn left on Russell Branch Parkway (becomes Cochran Mill Road). Continue about 1.5 miles until Samuel’s Mill Court; if you reach the Washington & Old Dominion Trail you have gone too far. Proceed on Samuel’s Mill Court, bearing right and up the hill. The pavement ends at a cul-de-sac, but continue up the hill on the unpaved road. The IAD radar dome will be on your left.
Coming from Sycolin Road, turn right on Cochran Mill Road (an unpaved road) and proceed about 1.8 miles. Soon after crossing the Washington & Old Dominion Trail, turn left on Samuel’s Mill Court. Proceed on Samuel’s Mill Court, bearing right and up the hill. The pavement ends at a cul-de-sac, but continue up the hill on the unpaved road. The IAD radar dome will be on your left.
Although you can approach very close, the dome itself is on a small parcel of secured government property and cannot be accessed by the public. Obey all signs and do not attempt to enter the FAA property.
J-50/Z-318 Joint Surveillance Radar in The Plains, Virginia
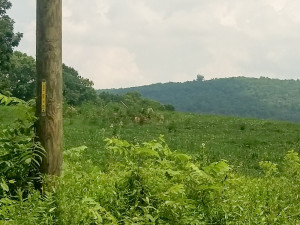
The J-50/Z-318 joint surveillance radar is maintained by the Federal Aviation Administration (FAA) and is located atop Signal Mountain northeast of The Plains, Virginia, in northeastern Fauquier County. It is about seven miles south of the border with Loudoun County.
The radar dome itself is very secluded and inaccessible, but can be viewed (at a great distance) from many of the surrounding highways. The closest view is most likely that from Bust Head Road (route 628) just north of its intersection with Hopewell Road (route 601). The actual entrance to the property is from Bull Run Mountain Road (route 629), but the dome itself is not visible from that location. The gate through which you might access the property is usually closed, and is clearly not intended for public access.
From Route 55 in The Plains, proceed north on Halfway Road. Immediately after crossing the railroad tracks, turn right on Hopewell Road (route 601). After about 2.5 miles, turn left on Bust Head Road (route 628) (unpaved). Proceed up the hill about 0.3 miles, and the J-50/Z-318 radar dome will be visible in the distance to your right.
From U.S. 50, proceed south on U.S. 15. about seven miles. Turn right on Waterfall Road (route 601) (later becomes Hopewell Road) and proceed about 5.5 miles. Turn right on Bust Head Road (route 628) (unpaved). Proceed up the hill about 0.3 miles, and the J-50/Z-318 radar dome will be visible in the distance to your right.
Unfortunately, there is no [legal] way that I am aware of to get closer to the J-50/Z-318 radar dome. Although it is possible to reach the entrance from Bull Run Mountain Road (route 629), it is gated. Do not attempt to enter the FAA property.
Bibliography
- National Centers for Environmental Information. “NEXRAD.” Link.
- National Centers for Environmental Information. “TDWR.” Link.
- Wikipedia.org. “Guglielmo Marconi.” Link.
- Wikipedia.org. “History of Radar.” Link.
- Wikipedia.org. “Joint Surveillance System.” Link.
- Wikipedia.org. “Radar.” Link.
- Wikipedia.org. “SAGE Radar Stations.” Link.